Thiosemicarbazones and their antimycobacterial effects
Thiosemikarbazony a jejich antimykobakteriální účinky
Antimykobakteriální účinky thiosemikarbazonů byly objeveny ve druhé polovině čtyřicátých let 20. století. Nejznámějším zástupcem těchto sloučenin je thioacetazon používaný v terapii tuberkulózy od přelomu čtyřicátých a padesátých let. Pro závažné vedlejší účinky se dnes používá jen málokdy. Tato práce pojednává o antimykobakteriálních účincích thiosemikarbazonů a N,N-dimethylthiosemikarbazonů odvozených od 5-alkyl-2-acetylpyrazinů. Některé z těchto sloučenin významně inhibovaly růst Mycobacterium tuberculosis H37Rv, ale pro svoji toxicitu nepostoupily do in vivo studií. Nicméně mohou být využity jako modelové sloučeniny pro studium mechanismů antimykobakteriálních účinků thiosemikarbazonů.
Klíčová slova:
tuberkulóza – thiosemikarbazony acetylpyrazinů – antimykobakteriální účinky
Authors:
Veronika Opletalová; Jan Doležel
Authors place of work:
Charles University in Prague, Faculty of Pharmacy in Hradec Králové, Department of Pharmaceutical Chemistry and Drug Analysis
Published in the journal:
Čes. slov. Farm., 2013; 62, 78-83
Category:
Původní práce
Summary
Antimycobacterial effects of thiosemicarbazones were discovered in the late 1940s. The best known representative of these compounds is thioacetazone that has been used in the therapy of tuberculosis since the turn of the 1940s and 1950s. At present, it is used only rarely since it exhibits severe side effects. This paper deals with the antimycobacterial effects of thiosemicarbazones and N,N-dimethylthiosemicarbazones derived from 5-alkyl-2-acetylpyrazines. Some of these compounds displayed high inhibition of the growth of Mycobacterium tuberculosis H37Rv, but were excluded from the in vivo studies due to their cytotoxic effects. Nonetheless, they can be used as model compounds for studying the mechanisms of antimycobacterial action of thiosemicarbazones.
Keywords:
tuberculosis – thiosemicarbazones of acetylpyrazines – antimycobacterial effects
Introduction
Tuberculosis is a very old disease. It is thought that the progenitor of the Mycobacterium tuberculosis complex arouse from a soil bacterium and that the human bacillus may have been derived from the bovine form following domestication of cattle approx. 10 000 years ago1– 3). Over the past 100 years, tuberculosis (TB) has probably killed 100 million people4). According to WHO Global Tuberculosis Report 2012, there were an estimated 8.7 million new cases of tuberculosis and 1.4 million died from TB in 2011, despite the availability of treatment that will cure most cases of TB5). Increased incidence of infections caused by the M. tuberculosis and M. avium complex in HIV-infected individuals6, 7) as well as evolution of multidrug-resistant tuberculosis (MDR-TB) and extensively drug-resistant tuberculosis (XDR-TB) present a serious problem8– 11). Moreover, the last first-line antimycobacterial agent – rifampicin 1 (RIFADIN®, Fig. 1) – was introduced more than 40 years ago12). In the end of 2012, bedaquiline 2 (SIRTURO®, Fig. 1) was approved by the FDA through an accelerated approval process only for patients who have multidrug-resistant TB, which can require up to 2 years of treatment. Phase III in MDR-TB patients are currently under way and may take 5 years. Only then the full potential of bedaquiline can be evaluated13). Thus, more-effective vaccines, diagnostic tools, drugs and therapeutic regimes are urgently needed14–16).
Antimycobacterial effects of thiosemicarbazones were discovered in the 1940s17) and resulted in a rapid introduction of thioacetazon (TAZ) 3 (CONTEBEN®, Fig. 2) into the therapy of tuberculosis19, 20). In spite of frequent side effects, TAZ may still be considered for the treatment of new cases if there is a lack of other antituberculous drugs and for the management of MDR-TB20). Several analogous thiosemicarbazones 4–5 (Fig. 2) have recently been studied21–24).
The unsubstituted acetylpyrazine thiosemicarbazone 6a (Fig. 3) has been known since 195225). Almost 50 years later, its congeners modified in the thiosemicarbazide part of the molecule 6b–6s and 7a–7g (Fig. 3) were studied as potential antituberculous drugs. Based on in vitro results, compound 6h was chosen for further testing on mice. Its potency was the same or even better than that of isoniazid and ethambutol. The best results were obtained with the dose of 5 mg/kg. The acute toxicity value (LD50) after per os administration was 90 mg/kg, and only small organic changes were observed26).
Within our studies aimed at finding new antimicrobial drugs, we have prepared and evaluated thiosemicarbazones 8a–8h and N,N-dimethylthiosemicarbazones 9a–9h alkylated on the pyrazine ring. The compounds exhibited iron chelating, antitumor and antifungal activities27). Thiosemicarbazones 8i and 9i derived from acetophenone were prepared for comparison (Fig 4).
The present paper deals with antimycobacterial effects of these compounds.
Experimental part
Primary screening of all compounds was conducted at 6.25 μg/ml against Mycobacterium tuberculosis H37Rv (ATCC 27294) in the BACTEC 12B medium using the Microplate Alamar Blue Assay (MABA)28). Compounds exhibiting fluorescence were tested in the BACTEC 460-radiometric system28). Compounds demonstrating at least 90% inhibition in the primary screen were re-tested at lower concentrations against M. tuberculosis H37Rv to determine the actual minimum inhibitory concentration (MIC) in the MABA. The MIC is defined as the lowest concentration effecting a reduction in fluorescence of 90% relative to controls.
The compounds that exhibited promising antimycobacterial activity were tested for cytotoxicity (IC50) in VERO cells at concentrations less than or equal to 10 times the MIC for M. tuberculosis H37Rv. After 72-h exposure, viability was assessed on the basis of cellular conversion of 1-(4,5-dimethylthiazol-2-yl)-2,5-diphenyltetrazolium (MTT) into a formazan product using the Promega CellTiter 96 Non- -radioactive Cell Proliferation Assay.
The selectivity index (SI) was then calculated as the ratio of the measured IC50 in VERO cells to the MIC described above. Generally, requirements for moving compound into in vivo testing include: MIC ≤ 6.25 μg.ml-1 and an SI ≥ 10.
The results of antimycobacterial and cytotoxicy assays are summarized in Table 1.
Results and discussion
Thiosemicarbazones 8a–8h exhibited high inhibition of M. tuberculosis. The lowest MIC (0.39 μg/ml) was observed with (2E)-2-[1-(5-butylpyrazin-2-yl)ethylidene] hydrazinecarbo thioamide 8d. N,N-dimethylthiosemicarbazones were less potent except for 9g and 9h which displayed potency similar to that of thiosemicarbazone 8d. It indicates that the character of the alkyl is important. In both series longer non-branched alkyls seem to be preferable for the antimycobacterial activity. This is in agreement with a recent study performed with ring substituted benzaldehyde thiosemicarbazones29). Regarding lipophilicity, N,N-αdimethylation results in approx. a twofold increase of log K values determined experimentally by means of HPLC27). All compounds with MIC ≤ 3.13 μg/ml have log K in the range 1.02–1.72 for thiosemicarbazone series and 2.20–2.62 for N,N-dimethylthiosemicarbazones.
Due to high cytotoxicity (low SI) none of the studied compounds was chosen for in vivo testing. Nonetheless, they can serve as tools to study mechanisms of action of thiosemicarbazones.
It is known that TAZ is a prodrug. In vivo it undergoes oxidative activation by flavin monooxygenase EthA, the enzyme that bioactivates also ethionamide 10 (TRECATOR®) and tiocarlide 11 (ISOXYL®) (Fig. 5)30, 31).
TAZ is also activated by human monooxidases FMO, FMO2.1 and FMO3 which may decrease the availability of the prodrug to the mycobacteria. The resulting metabolites – sulfenic acid 12 and carbodiimide 13, but not sulfinic acid 14 (Fig. 6) – react with glutathione (GSH) and may contribute the cytotoxicity of TAZ30–32).
Mycobacteria do not produce GSH but make mycothiol (MSH) which is essential for the growth of M. tuberculosis. MSH, like GSH, protects the cell against oxidative damage and electrophilic toxins. Metabolites of TZA that react with GSH should react with MSH in a similar manner thus lowering MSH concentration within the mycobacterial cell and sensitizing it to oxidative damage30).
It has also be found that TAZ inhibits cyclopropanation of cell wall mycolic acids in mycobacteria by inhibition of the enzymes belonging to CMAS (Cyclopropanating Mycolic Acids Synthases) family33, 34). A computational analysis showed that TAZ fits well at the active site of cyclopropane mycolic acid synthases CmaA1and CmaA2. Moreover, TAZ metabolites – TAZ-carbodiimide 13 and TAZ-sulfinic acid 14 (Fig. 5) – may also bind at the active site by NH-π interactions analogous to TAZ. If these hypotheses are validated experimentally, new knowledge about the TAZ binding site will be obtained35).
Other enzymes of CMAS family are also inhibited by TAZ even at extremely low doses with an exception of methoxy mycolic acid synthase MmaA4. Mutations in the mmaA4 gene make the mycobacteria resistant to TAZ. According to Alahari et al., activation of TZA by EthA itself is not sufficient, and TAZ must be further activated by MmaA4 to get the active form capable to induce growth arrest of mycobacteria34). However, this hypothesis is not supported by Grzegorzewicz et al.36).
Many other enzymes, especially those belonging to the fatty acid synthase (FAS ) system, have recently been studied as new drug targets37–39). M. tuberculosis possesses not only FAS-II monofunctional enzymes (specific for prokaryotes and organelles), but also mega-enzyme FAS-I (a multifunctional enzyme found mainly in eukaryotes). These two systems are engaged in the synthesis of normal chain-length fatty acids together with specific long-chain mycolic acids40). Moreover, M. tuberculosis encodes more that 60 adenylating enzymes (AE) that are essential for virulence. Development of potent and selective AE inhibitors represents another strategy in the fight against pathogenic mycobacteria41–43). Let’s hope that all these efforts will finally lead to more potent and safe antituberculous agents.
Acknowledgment
Antimycobacterial data were provided by the Tuberculosis Antimicrobial Acquisition and Coordination Facility (TAACF – http://www.taacf.org) through a research and development contract with the U.S. National Institute of Allergy and Infectious Diseases.
Received 11 December 2012 / Accepted 18 Februar 2013
Assoc. Prof. RNDr. Veronika Opletalová, Ph.D., J. Doležel
Charles University in Prague, Faculty of Pharmacy in Hradec Králové,
Department of Pharmaceutical Chemistry and Drug Analysis
Heyrovského 1203, 500 05 Hradec Králové, Czech Republic
e-mail: veronika.opletalova@faf.cuni.cz
Zdroje
1. Cole S. T., Brosch R., Parkhill J., Garnier T., Churcher C., Harris D., Gordon S. V., Eiglmeier K., Gas S., Barry III C. E., Tekaia F., Badcock K., Basham D., Brown D., Chillingworth T., Connor R., Davies R., Devlin K., Fettwell T., Gentles S., Hamlin N., Holroyd S., Hornsby T., Jagels K., Krogh A., McLean J., Moule S., Murphy L., Oliver K., Osborne J., Quail M. A., Rajandream M.-A., Rogers J., Rutter S., Seeger K., Skelton R., Squares R., Squares S., Sulston J. E., Taylor K., Whitehead S., Barrell B. G. Deciphering the biology of Mycobacterium tuberculosis from the complete genome sequence. Nature 1998; 393, 537–544.
2. Young D. B. Blueprint for the white plague. Nature 1998; 393, 515–516.
3. Tripathi R. P., Tewari N. T., Dwivedi N., Tiwari V. K. Fighting Tuberculosis: An old disease with new challenges. Med. Res. Rev. 2005; 25, 93–131.
4. Frieden T. R., Sterling T. R. Munsiff S. S., Watt C. J., Dye C. Tuberculosis. Lancet 2003; 362, 887–899.
5. World Health Organization. Global Tuberculosis Report 2012. http://www.who.int/tb/publications/global_report/en/ (11. 2. 2013)
6. Biava M., Porreta G. C., Deidda D., Pompei R. New trends in the development of antimycobacterial compounds. Infect. Disord.: Drug Targets 2006; 6, 159–182.
7. Kaufmann S. H. E. Tuberculosis and AIDS: A devilish liaison. Drug Discovery Today 2007; 12, 891–893.
8. Mukherjee J. S., Rich M. L., Socci A. R., Joseph J. K., Viru F. A., Shin S. S., Furin J. J., Becerra M. C., Barry D. J., Kim J. Y., Bayona J., Farmer P., Smith Fawzi M. C., Seung K. J. Programmes and principles in treatment of multidrug-resistant tuberculosis. Lancet 2004, 363, 474–481.
9. Kushwaha S. K., Shakya M. J. Protein interactions network analysis – Approach for potential drug target identification in Mycobacterium tuberculosis. J. Theor. Biol. 2010; 262, 284–294.
10. Minion J., Gallant V., Wolfe Y., Jamienson F., Long R. Multidrug and extensively drug resistant tuberculosis in Canada 1997–2008: Demographic and disease characteristics. PLoS One 2013; 8, e53466.
11. Donald P. R., van Helden P. D. The global burden of tuberculosis: Combating drug resistance in difficult times. N. Engl. J. Med. 2009, 360, 2393–2395.
12. Sensi P. History of development of rifampin. Rev. Infect. Dis. 1983; 5(Suppl. 3), S402–406.
13. Cohen, J. Approval of novel TB drug celebrated: With restraint. Science 2013; 339, 130.
14. Abu-Raddad, L. J., Sabatelli, L., Achtenberg, J. T., Sugimoto, J. D., Longini, I. M., Jr., Dye, C., Halloran, M. E. Epidemiological benefits of more-effective tuberculosis vaccines, drugs, and diagnostics. Proc. Natl. Acad. Sci. U. S. A. 2009; 106, 13980–13985.
15. Ginsberg A. M. Drugs in development for tuberculosis. Drugs 2010; 70, 2201–2214.
16. Ma Z., Lienhardt C., McIIIeron H., Nunn A. J., Wang X. Global tuberculosis drug development pipeline: The need and the reality. Lancet 2010; 375, 2100–2119.
17. Domagk G., Behnisch R., Mietzsch F., Schmidt H. Naturwissenschaften 1946; 33, 315.
18. Hantschman L., Werner R. Zu Kombinations Behandlung der Lungentuberkulose mit Conteben und PAS (Combined conteben and PAS therapy of pulmonary tuberculosis). Arztl. Wochensc. 1950; 5, 525–528.
19. Brecke F., Bohm, F. Zwei Jahre Conteben in Heilstätte (Two years´ sanatorium use of conteben). Hippokrates 1950; 21, 188–193.
20. Abate G., Koivula T., Hoffner S. E. In vitro activity on mycobacterial species belonging to the Mycobacterium tuberculosis complex. Int. J. Tuber. Lung Dis. 2002; 6, 933–935.
21. Bermudez L. F., Reynolds R., Kolonoski P., Aralar P., Inderlied C. B., Young L. S. Thiosemicarbazol (thiacetazone-like) compound with activity against Mycobacterium avium in mice. Antimicrob. Agents Chemother. 2003; 47, 2685–2687.
22. Bermudez L. F., Kolonoski P., Seitz L. E., Petrofsky M., Reynolds R., Wu M., Young L. S. SRI-268, a thiosemicarbazole, in combinatiom with mefloquine and moxifloxacin for treatment of murine Mycobacterium avium complex disease. Antimicrob. Agents Chemother. 2004; 48, 3556–3558.
23. Shahab F. M., Kobarfard F., Dadashzadeh S. Simultaneous determination of a new antituberculosis agent KBF-611 and its deacetylated metabolite in mouse and rabbit plasma by HPLC. Arch. Pharmacal Res. 2009; 32, 1453–1460.
24. Shahab F. M., Kobarfard F., Shafaghi B., Dadashzadeh S. Preclinical pharmacokinetics of KBF-611, a new antituberculosis agent in mice and rabbits, and comparison with thiacetazone. Xenobiotica 2010; 40, 225–234.
25. Kushner S., Dalalian H., Sanjurjo J. L., Bach F. L., Jr., Safir S. S., Smith V. K., Jr., Williams J. H. Experimental chemotherapy of tuberculosis. II. The synthesis of pyrazinamides and related compounds. J. Am. Chem. Soc. 1952; 74, 3617–3621.
26. Milczarska B., Foks H., Trapkowski Z., Milzynska-Kolaczek A., Janowiec M., Zwolska Z., Andrzejczyk Z. Studies on pyrazine derivatives. XXXII. Synthesis and tuberculostatic activity of acetylpyrazine thiosemicarbazone derivatives. Acta Pol. Pharm. 1998, 55, 289–295.
27. Opletalova V., Kalinowski D. S., Vejsova M., Kunes J., Pour M., Jampilek J., Buchta V., Richardson D. R. Identification and characterization of thiosemicarbazones with antifungal and antitumor effects: Iron chelation mediating cytotoxic activity. Chem. Res. Toxicol. 2008; 21, 1878–1889.
28. Collins L., Franzblau S. G. Microplate alamar blue assay versus BACTEC 460 system for high-throughput screening of compounds against Mycobacterium tuberculosis and Mycobacterium avium. Antimicrob. Agents Chemother. 1997; 41, 1004–1009.
29. Coxon G. D., Craig D., Corrales R. M., Vialla E., Gannoun-Zaki L. Synthesis, antitubercular activity and mechanism of resistance of highly effective thiacetazone analogues. PLoS One 2013; 8, e53162.
30. Qian L., Ortiz de Montellano P. R. Oxidative activation of thiacetazone by the Mycobacterium tuberculosis flavin monooxygenase EtaA and human human FMO1 and FMO3. Chem. Res. Toxicol. 2006; 19, 443–449.
31. Dover L. G., Alahari A., Gatraud P., Gomes J. M., Bhowruth V., Reynolds R. C., Besra G. S., Kremer L. EthA, a common activator of thiocarbamide-containing drugs acting on different mycobacterial targets. Antimicrob. Agents Chemother. 2007; 51, 1055–1063.
32. Francois A. A., Nishida C. R., Ortiz de Montellano P. R., Phillips I. R., Shephard E. A. Human flavin-containing monooxygenase 2.1 catalyzes oxygenation of antitubercular drugs thiacetazone and ethionamide. Drug Metab. Dispos. 2009; 37, 178–186.
33. Alahari A., Trivelli X., Guerardel Y., Dover L. G., Besra G. S., Sacchettini J. C., Reynolds R. C., Coxon G. D., Kremer L. Thiacetazone, an antitubercular drug that inhibits cyclopropanation of cell wall mycolic acids in mycobacteria. PLoS One 2007; 12, e1343.
34. Alahari A., Alibaud L., Trivelli X., Gupta R., Lamichhane G., Reynolds R. C., Bishai W. R., Guerardel Y., Kremer L. Mycolic acid methyltransferase, MmaA4, is necessary for thiacetazone susceptibility in Mycobacterium tuberculosis. Mol. Microbiol. 2009; 71, 1263–1277.
35. Banerjee D., Bhattacharyya R. Isoniazid and thioacetazon may exhibit antitubercular activity by binding directly with the active site of mycolic acid cyclopropane synthase: Hypothesis based on computational analysis. Bioinformation 2012; 8, 787–789.
36. Grzegorzewicz A. E., Korduláková J., Jones V., Born S. E., Belardinelli J. M., Vaquié A., Gundi V. A., Madacki J., Slama N., Laval F., Vaubourgeix J., Crew R. M., Gicquel B., Daffé M., Morbidoni H. R., Brennan P. J., Quémard A., McNeil M. R., Jackson M. A common mechanism of inhibition of the Mycobacterium tuberculosis mycolic acid biosynthetic pathways by isoxyl and thiacetazone. J. Biol. Chem. 2012; 287, 38434–38441.
37. Bellardinelli J. M., Morbidoni H. R. Mutations in the essential FAS II -hydroxyacyl ACP dehydratase complex confer resistance to thiacetazone in Mycobacterium tuberculosis and Mycobacterium kansasii. Mol. Microbiol. 2012; 86, 568–579.
38. Singh, V., Mani, I., Chaudhary, D. K., Somvanshi, P. The β-ketoacyl-ACP synthase from Mycobacterium tuberculosis as potential drug target. Curr. Med. Chem. 2011; 18, 1318–1324.
39. Rosado L. A., Caceres R. A., de Azevedo W. F., Jr., Basso L. A., Santos D. S. Role of serine 140 in the mode of action of Mycobacterium tuberculosis β-ketoacyl-ACP reductase (MabA). BMC Res. Notes 2012; 5, 526.
40. Cantaloube S., Veyron-Churlet R., Haddache N., Daffé M., Zerbib D. The Mycobacterium tuberculosis FAS-II dehydratases and methyltransferases define the specificity of the mycolic acid elongation complexes. PLoS One 2011; 6, e19564.
41. Arora P., Goyal A., Natarajan V. T., Rajacumara E., Verma P., Gupta R., Yousuf M., Trivedi O. A., Mohanty D., Tyagi A., Sankaranarayanan R., Gokhale R. S. Mechanistic and functional insight into fatty acid activation in Mycobacterium tuberculosis. Nat. Chem. Biol. 2009; 5, 166–173.
42. Mohanty D., Sankaranarayanan R., Gokhale R. S. Fatty acyl-AMP ligases and polyketides synthases are unique enzymes of lipid biosynthetic machinery in Mycobacterium tuberculosis. Tuberculosis 2011; 91, 448–455.
43. Duckworth B. P., Nelson K. M., Aldrich C. C. Adenylating enzymes in Mycobacterium tuberculosis as drug targets. Curr. Top. Med. Chem. 2012; 12, 766–796.
Štítky
Farmacie FarmakologieČlánek vyšel v časopise
Česká a slovenská farmacie
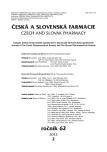
2013 Číslo 2
- Čokoláda podávaná v malých dávkách neškodí. Vědecky prokázáno!
- MUDr. Václav Šmíd, Ph.D.: Jaterní fibróza a iniciální stadia cirhózy jsou potenciálně vratné stavy
- Esenciální fosfolipidy v podpůrné léčbě jaterní steatózy asociované s metabolickou dysfunkcí
- FDA varuje před selfmonitoringem cukru pomocí chytrých hodinek. Jak je to v Česku?
Nejčtenější v tomto čísle
- Evaluation of the influence of sterilization method on the stability of carboxymethyl cellulose wound dressing
- Dr.h.c., prof. RNDr. Jozef Čižmárik, PhD. – sedemdesiatnik
- Prolegomenon of the Czech pharmacognosy: 21st century
- Fytoterapia v súčasnej medicíne